Translate this page into:
Safety evaluation and potential health implications of water from post-remediated lead-polluted areas of Zamfara State, Nigeria
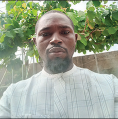
*Corresponding author: Dr. Michael Oko Odey, Department of Biochemistry, Faculty of Basic Medical Sciences, University of Calabar, Nigeria. michaelodey@unical.edu.ng
-
Received: ,
Accepted: ,
How to cite this article: Odey MO, Udiba UU, Adindu EA, Enyievi PB, Edu BC, Eteng MU, et al. Safety evaluation and potential health implications of water from post-remediated lead-polluted areas of Zamfara State, Nigeria. Calabar J Health Sci 2022;6:15-23.
Abstract
Objectives:
Dareta village in Zamfara state of Nigeria is one of the villages plagued with the lead (Pb) poisoning crisis of 2010. Dareta and neighboring villages were remediated by simple excavation of topsoil rich in Pb, and burying same in landfills. This study was embarked to assess the physicochemical parameters of some sources of water supply and possible health impacts on humans.
Material and Methods:
The village was imaginarily mapped into three sites; Residential Homes (Site 1), Markets and Village Squares (Site 2), and Farms with Irrigation Facilities (Site 3). From the three sites, a total of 30 water samples (10 shallow wells, 10 boreholes, and 10 stream/surface water) were randomly collected, according to availability. The physical parameters (Temperature, Conductivity, and Total Dissolved Solids [TDS]) were assessed in situ using a conductivity/TDS meter (model 44600.00, HACH, USA), the assessment of pH was also carried out in situ using an electronic pH meter, (pH 210 micro-processor, Hanna Instrument, USA). The evaluation of heavy metals concentration (Pb, cadmium [Cd], chromium [Cr], and manganese) was done using an Atomic Absorption Spectrophotometer (modelAA-6800, Schemadzu, Japan).
Results:
In groundwater (boreholes and wells), the mean temperature and conductivity were higher than the World Health Organization (WHO) safety levels for “drinking water,” while those of TDS and pH were within the limits. In surface water (streams), the assessed physical parameters were not higher than the WHO safety limits, while the concentrations of Pb, Cd, and Cr were above the drinking water guidelines. The risk implication in the human populations consuming the water was determined using the estimated average daily intake (EADI) and the target hazard quotient (THQ). The EADI for Pb, Cd, and Cr for all the water sources in both adult and children consumer populations exceeded the reference dose by US-EPA. The THQ for Pb, Cd, and Cr in both adult and children consumer populations were >1.
Conclusion:
These findings portend toxicity and increased hazards for the human populations that source their drinking water from the borehole, well, and stream in this village.
Keywords
Safety evaluation
Physicochemical parameters
Water sources
Toxicity potentials
Target hazard quotient
Estimated daily intake
INTRODUCTION
Environmentally related pollution and hazards associated with public health are on the rise globally. The World Health Organization (WHO) has reported that globally, over 24% of causative agents for diseases are a result of factors that are related to the environment.[1] Factors of the environment that are mostly considered in this respect are pollution of soil, water, and air, which are consequent to exposure potentially, to biological, and chemical entities that are carried in the air in form of particulates, cancer-causing agents, harmful metals, and substances that can injure or disrupt endocrine glands.[2] Humans have encouraged the spread and buildup of these unwanted substances in our environment. Toxic heavy metals have considerably added to the pollution of our environments and have been given the utmost attention in relation to their origins and effects, particularly those that are seen to be beneficial or harmful or show a level of geochemical abundance.[3]
The presence of both ionized and unionized metals and their alloys in groundwater can pose a high level of threat to human health and other biotic and abiotic (ecological) systems. The forms in which the chemical pollutants exist determine and affect their solubility, movement, and the extent of toxicity in water bodies below the earth’s surface. The form of metal exists chemically depends greatly on the origin of the waste, the chemistry of the soil, and the groundwater associated with it. Water bodies on the surface of the earth and groundwater may be polluted with heavy metals from discharges containing these metals or by coming in contact with soils, wastes from mining, debris, and sludge polluted with these metals. The sources of the pollutants affect the heterogeneity of polluted sites on a large or small scale, while changes in the concentrations of the pollutants and matrices affect the dangers associated with pollutions related to metals and the options to be taken for treatment.[4]
Heavy metals consist of a particular class of pollutants of interest, gotten from both man-made and natural sources, and have the ability to penetrate interfaces and environmental matrices.[5,6] Toxicity related to heavy metals pollution and their rising environmental levels has necessitated a lot of research effort geared toward assessing the health effects related to their presence in potential sources of drinking water.[7-9] Methods that showed the possible hazards of polluted water and foods to the health of humans, by intake through the mouth have been on the basis of the “estimated average daily intake” (EADI) and the “target hazard quotient” (THQ).[10-12] The THQ-related method of hazard assessment provides a more dependable and useful pointer to the hazard level related to contact or contaminants-related exposure.[10,13]
Dareta village such as several rural and semi-urban communities in Nigeria lacks the provision of municipal pipe-born water, in addition to the intense lead (Pb) pollution situation in these communities. As a result, the rise in direct use and abuse of water bodies at both underground and surface levels, coupled with other factors such as illegal mining of Gold-ore and its processing, rise in precipitation, and land use activity, which add to susceptibility to pollutants of these water bodies, are important reasons to worry about when considering water sources and usage in Dareta communities. The present study was directed towards assessing the level of fitness of sources of water and possible human health effects to Dareta communities in Zamfara state of North-western Nigeria.[14,15]
MATERIAL AND METHODS
Sampling sites
This research was conducted to cover the entire village, including residential areas, markets and village squares, and farms with irrigation facilities in “Dareta village of Anka local government area of Zamfara state, North-western Nigeria.” Udiba et al.[16] had previously reported that “Dareta village is located on 12°06'30'' N and 5°56'00'' E with a total area of about 2,746 sq km and a total human population of about 142, 280, of which about 20% are children under age 5 years [Figure 1]. The village is populated primarily by Hausa and Fulani. Not until recently, following the discovery of Gold mines, the major activity of the people of Dareta village was farming. Recently artisanal mining activities engaged a large percentage of the population. Dareta, Abare, Tungandaji, Sunke, Tungar guru, Duza, and Bagega are the major villages where the 2010 outbreaks of Pb poisoning occurred.” Irrespective of the large and busy industrial activities, there is the absence of modern infrastructures, such as municipal water supply in the study area. Consequent to this, the people seek and depend strongly on alternative water supplies, such as surface water (stream) and groundwater (shallow wells and boreholes) to meet their water needs. Dareta village in Zamfara state, Nigeria is one of the villages plagued with Pb poisoning in 2010. A remediation exercise was carried out in 2010, in an attempt to mitigate the poisoning situation in Dareta and other villages. This process of remediation or clean-up involved the excavation of the topsoil with high Pb content and burying same in landfills. Zamfara state is prone to high wind and water erosion, and there was a tendency for re-exposure of these contaminated soils, with the soil Pb carried by wind or water (due to erosion) into available water sources. This work was carried out in Dareta village, 7 years post-remediation, to check the effectiveness of the cleanup exercise.
![Anka local government area showing Dareta; the study area with the sampling points.[16]](/content/111/2022/6/1/img/CJHS-6-015-g001.png)
- Anka local government area showing Dareta; the study area with the sampling points.[16]
Collection and analysis of samples
Collection of surface and groundwater samples for physicochemical analysis
The village was imaginarily mapped into three sites; Residential Homes (Site 1), Markets and Village Squares (Site 2), and Farms with Irrigation Facilities (Site 3). From the three sites, a total of 30 water samples (10 shallow wells, 10 boreholes, and 10 stream/surface water) were randomly collected, according to availability. Water samples were randomly collected in June 2017, using acid-washed polypropylene containers. (n = 10/water source, i.e., 10 samples from shallow wells (25–30 m deep), 10 samples from boreholes (40–300 m deep, depending on soil type), and 10 samples from streams across the study area, using plastic drawers and from surface water bodies by simply scooping, using a plastic bucket, (at 350–400 m apart; based on accessibility, along with the courses of the rivers traversing the village), into 4 L `acid washed polypropylene containers. For the collection of water samples from the well, the fetcher was washed thoroughly and rinsed with clean and distilled water respectively, before it was used to fetch water from the well. The water was then carefully turned into a sterilized plastic container for elemental analysis, while part was transferred into a sterilized 200 m beaker for in situ physicochemical analysis. To collect borehole water samples, the outlets (taps) were opened and some water was allowed to pour out before carefully pouring into sterilized plastic containers for heavy metal analysis and a beaker for in situ physicochemical analysis.
Physicochemical assay
The physicochemical parameters assayed include; EC, TDS, pH, and temperature. The Temperature, EC, and TDS were assessed in situ using a conductivity/TDS meter (model 44600.00 HACH Company, USA). The determination of the pH was done electronically in situ using pH meters (pH 210 mp, Hanna instrument, USA).[16] The handling of the samples was as reported by Udiba et al.[16] and was used subsequently for the assessment of heavy metals in the samples.
Preparation of water samples for heavy metals analysis
The preparation of water samples for heavy metals analysis was according to the methods reported by Prasad et al.,[17] “The sample was thoroughly mixed, 20 ml was transferred into a conical flask, 10 ml conc. nitric acid was added and brought to slow-boiling on an evaporating plate to the lowest volume (10–20 ml). Nitric acid was added as necessary until digestion was completed as shown by the light color/clear solution. The solution was not allowed to get dried during digestion. The digest was filtered into a 50 mL volumetric flask and made up to the mark with distilled water. The digested water samples were used for the determination of Pb, cadmium (Cd), chromium (Cr), and manganese (Mn), using AAS, (model AA-6800 Shimadzu, Japan). The heavy metals concentrations in the samples were assessed by comparison of absorbance with those of standards for the elements.[17]
Risk assessment
EADI
The EADI was determined by multiplying the average residual heavy metal concentration (mg/L) by the estimated water consumption rate (liters/day).
Micheal et al.,[4] USEPA[18] had reported that “Residual concentration is the concentration of metal in a water sample (in milligrams per liters), a conservative ingestion rate of water per day being estimated to be 1 L for a 10 kg child and 2 L for an adult with bodyweight set at 70 kg for the adult population.” The EADI was compared to reference dose (RfD) which “represents the threshold exposure below which it is unlikely for even sensitive populations to experience adverse health effects.”[4,18,19] Further clarified that “RfD – reference dose; the term used in the evaluation of the risk of toxic effects of various chemicals including metals on humans.[19] The RfD is defined by USEPA as an estimate (with uncertainty spanning perhaps an order of magnitude) of a daily exposure to the human population (including sensitive subgroups) that is likely to be without an appreciable risk of deleterious effects during a lifetime.”
The THQ
This is the ratio of the EADI (mg/kg body weight/day) of a chemical to an RfD, mg/kg/day, defined as the Maximum Tolerable Daily Intake of a specific metal that does not result in any deleterious health effects (Eq. 2):
If the value of THQ is above 1 (i.e., THQ >1), this means that the exposed population via the consumption of contaminated water is likely to experience obvious deleterious effects. The probability of the hazard risk in humans and the THQ is directly proportional.
For the risk assessment of multiple metals contained in water samples, a total hazard index (THI) was employed by summing all the calculated THQ values of metals as described in Eq. (3).[18]
Where THQi is the THQ of an individual element of metals, THI is the THI for all the four (4) metals determined in this study. The determination was done in quadruplets.
Statistical analysis
Data obtained were presented as mean ± SD and tested for normal distribution using Shapiro–Wilk’s test and homogeneity of variance was tested with Levene’s test. A t-test was used to compare the levels of metals in ground and surface water samples, while one-way ANOVA was performed to test significant differences between the three samplings (Residential areas, markets, and village squares, and farms with irrigation facilities) sites and values were considered significant at P < 0.05.
RESULTS
Physicochemical parameters of ground (wells) water samples
The results of the physicochemical parameters of water (ground and surface), randomly sampled from residential areas, markets, and village squares, and farms with irrigation facilities in Dareta village are presented in [Table 1]. The results show that the mean temperature of the groundwater was 28.34 ± 206°C for the well and 30.19 ± 0.29°C for the borehole. The stream water has a mean temperature of 30.06 ± 0.48°C. All the mean temperature values were above the WHO permissible value of 25°C. The average pH values were 6.69 ± 0.33 for well, 6.66 ± 0.41 for borehole, and 6.60±1.04 for stream and were all within the permissible pH range set by the WHO and NSDWQ for domestic water. The mean electrical conductivity (µs/cm) was 631.00 ± 141.13 for well, 660.70 ± 236.41 for borehole, and 326.00 ± 51.44 for stream. All the mean values were higher than the WHO set value of 500 but lower than the NSDWQ set value of 1000. The mean total dissolved solids (TDS) were 290.20 ± 63.09 mg/l for well, 332.50 ± 116.89 mg/l for borehole, and 174.60 ± 30.40 mg/l for stream. They were all lower than the set standards. Statistical analysis showed that the TDS of groundwater was significantly (P < 0.01) correlated with conductivity (r = 0.823).[4,20]
Water source | Temperature (°C) | pH | Electrical conductivity (μs/cm) | TDS (mg/l) |
---|---|---|---|---|
Wells | 28.34±2.06 | 6.69±0.33 | 631.00±141.13 | 290.20±63.09 |
Boreholes | 30.19±0.29 | 6.66±0.41 | 660.70±236.41 | 332.50±116.89 |
Streams | 30.06±0.48 | 6.60±1.04 | 326.00±51.44 | 174.60±30.40 |
WHO std | 25 | 6.5-8.5 | 250 | 500 |
NSDWQ | - | 6.5-8.5 | 1000 | 500 |
Heavy metals concentration in water sources from Dareta village, Zamfara State, Nigeria
The mean values of heavy metals concentration in a shallow well, borehole, and stream waters from Dareta village of Zamfara State, Nigeria are presented in [Table 2]. The results showed that the values recorded for Pb (mg/l), 1.27 ± 0.20 for well, 0.99 ± 0.26 for borehole, and 0.88 ± 0.38, were significantly increased compared to the set standards. The mean Cd values were 0.04 ± 0.01 (mg/l) in both well, borehole, and stream; this value was higher than the standard of 0.005 set by WHO, Canadian, USEPA, NESREA, and SON. The mean Cr values were 0.14 ± 0.03 mg/l for well, 0.14 ± 0.06 mg/l for borehole, and 0.17 ± 0.04 mg/l for stream. These were all higher than the set standard of 0.005. The mean Mn values were 0.05 ± 0.01 mg/l for well, 0.05 ± 0.01 mg/l for borehole, and 0.07 ± 0.01 mg/l for stream.
Metal (mg/L) | Well | Borehole | Stream | WHO std | NESREA | USEPA | Canadian std | SON |
---|---|---|---|---|---|---|---|---|
Lead | ||||||||
1 | 1.23 (PG) | 0.02 (PG) | 0.86 (PG) | |||||
2 | 1.30 (PG) | 0.05 (PG) | 0.90 (RA) | |||||
3 | 1.21(RA) | 0.03 (PG) | 0.89 (RA) | |||||
4 | 1.33 (RA) | 0.06 (RA) | 0.87(Ir) | |||||
5 | 0.99 (RA) | 0.04 (RA) | 0.93 (Ir) | |||||
6 | 1.02 (RA) | 0.05 (RA) | 0.83 (Ir) | |||||
7 | 1.27 (Ir) | 0.03 (RA) | 0.76 (Ir) | |||||
8 | 1.55 (Ir) | 0.03 (RA) | 1.01 (Ir) | |||||
9 | 1.22 (Ir) | 0.04 (Ir) | 0.89 (Ir) | |||||
10 | 1.57 (Ir) | 0.03 (Ir) | 0.89 (Ir) | |||||
Mean±SD | 1.27±0.20 | 0.99±0.26 | 0.88±0.34 | 0.001 | 0.01 | 0.015 | 0.01 | 0.01 |
Cadmium | ||||||||
1 | 0.03 (PG) | 0.02 (PG) | 0.04 (PG) | |||||
2 | 0.05 (PG) | 0.05 (PG) | 0.05 (RA) | |||||
3 | 0.04 (RA) | 0.03 (PG) | 0.03 (RA) | |||||
4 | 0.06 (RA) | 0.06 (RA) | 0.05 (Ir) | |||||
5 | 0.02 (RA) | 0.04 (RA) | 0.05 (Ir) | |||||
6 | 0.05 (RA) | 0.05 (RA) | 0.04(Ir) | |||||
7 | 0.03 (Ir) | 0.03 (RA) | 0.03 (Ir) | |||||
8 | 0.03 (Ir) | 0.03 (RA) | 0.05 (Ir) | |||||
9 | 0.05 (Ir) | 0.04 (Ir) | 0.02 (Ir) | |||||
10 | 0.05 (Ir) | 0.03 (Ir) | 0.06 (Ir) | |||||
Mean±SD | 0.04±0.01 | 0.04±0.01 | 0.04±0.01 | 0.003 | 0.005 | 0.005 | 0.005 | 0.003 |
Chromium | ||||||||
1 | 0.12 (PG) | 0.13 (PG) | 0.20 (PG) | |||||
2 | 0.14 (PG) | 0.15 (PG) | 0.15 (RA) | |||||
3 | 0.13 (RA) | 0.14 (PG) | 0.16 (RA) | |||||
4 | 0.16 (RA) | 0.16 (RA) | 0.20 (Ir) | |||||
5 | 0.14 (RA) | 0.15 (RA) | 0.15 (Ir) | |||||
6 | 0.16 (RA) | 0.12 (RA) | 0.17 (Ir) | |||||
7 | 0.15 (Ir) | 0.14 (RA) | 0.18 (Ir) | |||||
8 | 0.15 (Ir) | 0.16 (RA) | 0.16 (Ir) | |||||
9 | 0.13 (Ir) | 0.12 (Ir) | 0.14 (Ir) | |||||
10 | 0.15 (Ir) | 0.13 (Ir) | 0.15 (Ir) | |||||
Mean±SD | 0.14±0.03 | 0.14±0.06 | 0.17±0.04 | 0.050 | 0.005 | 0.1 | 0.05 | 0.05 |
Manganese | ||||||||
1 | 0.03 (PG) | 0.04 (PG) | 0.05 (PG) | |||||
2 | 0.07 (PG) | 0.06 (PG) | 0.08 (RA) | |||||
3 | 0.04 (RA) | 0.03 (PG) | 0.06 (RA) | |||||
4 | 0.05 (RA) | 0.07 (RA) | 0.07 (Ir) | |||||
5 | 0.06 (RA) | 0.04 (RA) | 0.05 (Ir) | |||||
6 | 0.04 (RA) | 0.05 (RA) | 0.07 (Ir) | |||||
7 | 0.05 (Ir) | 0.05 (RA) | 0.08 (Ir) | |||||
8 | 0.04 (Ir) | 0.06 (RA) | 0.06 (Ir) | |||||
9 | 0.06 (Ir) | 0.03 (Ir) | 0.08 (Ir) | |||||
10 | 0.05 (Ir) | 0.04 (Ir) | 0.06 (Ir) | |||||
Mean±SD | 0.05±0.01 | 0.05±0.01 | 0.07±0.01 |
Potential health risk assessment of heavy metal in well, borehole, and stream from Dareta village, Zamfara State, Nigeria
The assessment of potential health risks of heavy metals in well, borehole, and stream from Dareta village, Zamfara State, Nigeria for both adults and children is presented in [Table 3]. The EADI for both adult and children were higher than the RfD for all the metals. The EADI shows a higher risk of exposure to Pb across the three sources of water in children than in adults, with consequent higher EADI values than those of adults. The same trend was observed for Cd, Cr, and Mn. The total hazard quotient for Pb, Cd, and Cr were all above the threshold value of 1 for both adults and children. The hazard quotients were higher for children than adults. For an adult, the THQ for Cr was higher than that of Pb, and Pb higher than that of Cd. For children, the THQ for Cr in the well and borehole was higher than those of Pb in the three water sources, while that of Cr in the stream was less than the Pb values. The THQ for Cd was the lowest, compared to that Pb and Cr. However, the THQ for Mn in all the water sources for both adult and children were less than the threshold value of 1.[4,18,21]
Metal | Site | Mean (mg/L) | Intake per day (liters) | Adult weight (kg) | EADI | RfD (mg/kg/ day) USEPA (2001) | *THQ (EADI/ RfD) | Intake per day (litres) | Children weight (kg) | EADI | THQ |
---|---|---|---|---|---|---|---|---|---|---|---|
Pb | Well | 1.27±0.20 | 2 | 70 | 0.0363 | 0.00357 | 10.17 | 1 | 10 | 0.1270 | 35.57 |
Borehole | 0.99±0.26 | 2 | 70 | 0.0283 | 0.00357 | 7.93 | 1 | 10 | 0.0990 | 27.73 | |
Stream | 0.88±0.38 | 2 | 70 | 0.0251 | 0.00357 | 7.03 | 1 | 10 | 0.0880 | 24.65 | |
Cd | Well | 0.04±0.01 | 2 | 70 | 0.0011 | 0.001 | 1.10 | 1 | 10 | 0.0040 | 4.00 |
Borehole | 0.04±0.01 | 2 | 70 | 0.0011 | 0.001 | 1.10 | 1 | 10 | 0.0040 | 4.00 | |
Stream | 0.04±0.01 | 2 | 70 | 0.0011 | 0.001 | 1.10 | 1 | 10 | 0.0040 | 4.00 | |
Cr | Well | 0.14±0.03 | 2 | 70 | 0.0040 | 0.0003 | 13.33 | 1 | 10 | 0.0140 | 46.67 |
Borehole | 0.14±0.06 | 2 | 70 | 0.0040 | 0.0003 | 13.33 | 1 | 10 | 0.0140 | 46.67 | |
Stream | 0.17±0.04 | 2 | 70 | 0.0045 | 0.0003 | 15.00 | 1 | 10 | 0.0050 | 16.67 | |
Mn | Well | 0.05±0.01 | 2 | 70 | 0.0014 | 0.14 | 0.01 | 1 | 10 | 0.0050 | 0.04 |
Borehole | 0.05±0.01 | 2 | 70 | 0.0014 | 0.14 | 0.01 | 1 | 10 | 0.0050 | 0.04 | |
Stream | 0.07±0.01 | 2 | 70 | 0.0020 | 0.14 | 0.01 | 1 | 10 | 0.0070 | 0.05 |
DISCUSSION
One of the main interests of the sustainable development goals set by the United Nations (UN) is to increase access to improved and quality water supplies, especially in rural communities lacking such supplies.[14,19] This research investigated water quality and possible implications for human health, relying on shallow wells, boreholes, and surface water sources for both domestic and agricultural activities in Dareta Village of Zamfara state, Nigeria. This research was aimed at contributing useful information towards the provision of suitable and sustainable water supplies in these communities and the sub-region plagued with lead and other heavy metals pollution, due to illegal gold mining activities in the area. At present, there is no form of municipal water supply in the study area. Furthermore, there is an information gap on the quality of water sources in Dareta, Zamfara state, Nigeria.
Normal fluctuations in climate bring about variations in the temperature of water bodies. These variable changes could occur over a period of a day (24 h) in some bodies of water, and seasonally in some cases. Factors such as depth of water body, time of day, cloud cover and the flow, air circulation, latitude, time of day, season, and altitude affect surface water temperature. On the other hand, natural processes are affected by temperature, which invariably affects the concentration of many variables in water bodies. Chemical reaction rate, along with volatilization and evaporation generally increases with an increase in water temperature. Once the temperature of water changes, it can control other parameters including redox potential, pH, specific conductivity, and concentrations of dissolved oxygen as well as other dissolved gases such as CO2, H2S, N2, and CH4.[22,23] In the report of Dahunsi et al.,[23] “Surface waters are usually within the temperature range 0–30°C, although “hot springs” may reach 40°C or more. Groundwater usually maintains a fairly constant temperature which, for superficial aquifers, is normally close to the mean annual air temperature. However, deep aquifers have higher temperatures due to the earth’s thermal gradient.” In this research, the recorded mean groundwater temperature was slightly above the WHO recommended temperature. This however may be due to the seasonal changes during sample collection. The mean surface water temperature, however, was within the WHO recommended temperature for surface water.
The pH, which defines the basicity or acidity of water represents a state of balance between H+ and OH- of water. Chemically, pH usefully indicates an ionic balance in the water. The availability of certain nutrients or chemicals can be adversely affected by changes in pH. The pH of water is a key variable in the evaluation of water quality because of its influence on chemical and biological processes in the water, including those related to water treatment and supply.[24]
At a certain temperature, pH portends the intensity of the alkaline or acidic property of the solution, which is controlled by chemical compounds dissolved in the water, and other biochemical processes in the solution.[20] In this study, the recorded temperature for both ground and surface water was within the recommended values for potable domestic water.
This research revealed that surface water, shallow well and borehole water sources used in the study area are laden with a high concentration of heavy metals (Pb, Cd, and Cr) in ranges above those of WHO, NESREA, USEPA, SON, and Canadian permissible standards for drinking water. Concentrations of Mn in the various samples of water were within the permissible levels set by National and international drinking water guidelines. Furthermore, evaluation of probable risks, shown by EADI of metals for human consumer population indicated site-specificity in risks of heavy metals poisoning for different populations. This specificity trend may be a reflection of specificity in the vulnerability of surface and groundwater which is dependent on the interplay of prevailing human activities (such as mining) and natural factors at different sites. This position was in tandem with previous studies, documenting differential vulnerability of surface and groundwater contamination, depending on a number of factors such as amount of precipitation, higher infiltration, highly depleted protective cover, excavation, and complex land use activities.[25]
This research also recorded Pb, Cd, and Cr concentrations, which were higher than those of drinking water guidelines. These increased levels of toxic metals above the permissible levels for drinking water guidelines vividly indicated that these sources of water are not ideal and safe for domestic uses, especially for consumption, and could possibly lead to unintended health impacts on user populations because of the related health implications on humans, when exposed to toxic heavy metals.
The research also implicated the use of EADI and THQ in the evaluation of potential health implications of human exposure to toxic heavy metals in water, across various age ranges within the human population in the study area. The EADI of Pb and Cr in all the water sources in all the sampling sites indicated values higher than RfD for these heavy metals (Pb and Cr) intake, for the adult consumer population. For the children consumer population, the EADI via well, borehole, and surface water across all the sampling sites showed that Pb, Cd, Cr, and Mn EADI values exceeded the RfD intake for the child consumer population, except for Cd in the borehole from markets and village squares and Cd in streams from farms with irrigation facilities. Since “RfD is an estimate of safe daily exposure to the human population (including sensitive subgroups) during a lifetime,” it portends that both adult and children consumer populations in the study area face higher risks of Pb, Cd, and Cr toxicity via dietary intake, though this may be lower in children.
The values showed by THQ are suggested as an integral index for comparison of ingestion amount of pollutants, with a standard RfD, and have been widely used in the risk assessment of metals in contaminated food sources.[26] A THQ value below 1 means the exposed populations are unlikely to experience an obviously adverse effect, whereas a THQ above 1 means that there is a chance of non-carcinogenic effects, with an increasing probability as the values increase.
This study has demonstrated that the concentrations of heavy metals (Pb, Cd, and Cr) in well, borehole, and surface water samples commonly used for both domestic and agricultural activities in communities in Dareta exceeded the National and international drinking water guidelines suggesting that these water sources are contaminated and considered unsafe for human consumption. The increase in these heavy metals may have been responsible for the lead poisoning crisis of 2010, in which over 200 women and children died due to lead poisoning. Furthermore, at the time of sample collection, possible signs and symptoms such as low cognitive capacity, lack of concentration, children looking younger than their age, and other behavioral changes were observed. The potential human health risk assessment by evaluating the EADI and the targeted hazard quotient (THQ) for the child and adult populations indicated that the adult population in the study area consuming borehole, well and stream water are at risk of Pb and Cr toxicity, while the child population consuming water from these sources are at risk of Pb, Cd, and Cr toxicity. It is also worthy of note that in spite of the relative safety alluded to boreholes due to their relative depth, this study reported a significant increase in metal concentrations in water samples from shallow wells and boreholes. Since ground water quality issues are receiving widespread attention, in-depth and more extensive studies that provide information on groundwater vulnerability is recommended for the effective protection and management of groundwater quality.
CONCLUSION
This study revealed that the cleanup method adopted in the remediation of the Lead pollution effect in the study area was not effective on a long term basis. This is because the re-exposure of the Lead polluted soil to the surface may have contributed to the rather higher than normal level of the metals studied, especially in the surface water. Also, the method of remediation; by burying contaminated soil in land fills, that were already water-logged may have been responsible for the increased levels of these metals in ground water, through leeching of these metals into the underground water. It is imperative to adopt a more holistic remediation approach, such as phyto-remediation to completely remove Lead and its associated heavy metals from the polluted environment.
Declaration of patient consent
Patient’s consent not required as there are no patients in this study.
Financial support and sponsorship
Nil.
Conflicts of interest
There are no conflicts of interest.
References
- Environmental Pollution and Impact to Public Health; Implication of the Dandora Municipal Dumping Site in Nairobi, Kenya Nairobi, Kenya: United Nation Environmental Program; 2007. p. :153-9.
- [Google Scholar]
- Spatial and temporal variations in water and sediment quality of Ona River, Ibadan, Southwest Nigeria. Eur J Sci Resour. 2012;74:186-204.
- [Google Scholar]
- Drinking water quality and risk implications for community health: A case study of shallow water wells and boreholes in three major communities in Northern cross-river, Southern Nigeria. Hum Ecol Risk Assess Int J. 2018;24:427-44.
- [CrossRef] [Google Scholar]
- Heavy metal content and distribution in surface sediments of the Seyhan River, Turkey. J Environ Manage. 2011;92:220-9.
- [CrossRef] [PubMed] [Google Scholar]
- Risk assessment and toxic effects of metal pollution in two cultured and wild fish species from highly degraded aquatic habitats. Arch Environ Contaminat Toxicol. 2013;65:53-64.
- [CrossRef] [PubMed] [Google Scholar]
- Health risk assessment for exposure to some selected heavy metals via drinking water from Dadinkowa dam and river gombeabba in Gombe state, Northeast Nigeria. World J Anal Chem. 2016;4:1-5.
- [Google Scholar]
- Drinking water studies: A review on heavy metal, application of biomarker and health risk assessment (a special focus in Malaysia) J Global Health. 2015;5:297-310.
- [CrossRef] [PubMed] [Google Scholar]
- Health risk assessment of heavy metals in drinking water based on field measurement of exposure factors of Chinese people. Huan Jing Ke Xue. 2011;32:1329-39.
- [Google Scholar]
- Metal residues in flesh of edible blue crab, Callinecte samnicola, from a tropical coastal lagoon: Health implications. Hum Ecol Risk Assess. 2016;22:1708-25.
- [CrossRef] [Google Scholar]
- Human health risk assessment of trace metals in water from Quaiboe river estuary, Ibeno, Nigeria. J Environ Occup Sci. 2015;4:151.
- [CrossRef] [Google Scholar]
- Health risk assessment of heavy metals and their source apportionment in drinking water of Kohistan region, Northern Pak. Microchem J. 2011;98:334-43.
- [CrossRef] [Google Scholar]
- Heavy metal contamination of African Cat Fish (Clarias gariepinus) from industrial effluent and domestic waste-contaminated rivers and home bred sources in Zaria, Nigeria. Afr J Biomed Res. 2020;23:101-5.
- [Google Scholar]
- Gross pathology, physiological and toxicological responses in relation to metals and persistent organic pollutants (POPs) burden in tilapia species from Ogun River, Nigeria. Mar Environ Res. 2017;129:245-57.
- [CrossRef] [PubMed] [Google Scholar]
- Proposed APLIE method for groundwater vulnerability assessment in karst-phreatic aquifer, Shandong Province, China: A case study. Environ Earth Sci. 2016;75:112-9.
- [CrossRef] [Google Scholar]
- Post remediation assessment of contaminants levels in soil, Dareta village, Zamfara, Nigeria. J Trends Adv Sci Eng. 2012;4:70-9.
- [Google Scholar]
- Ground water quality evaluation near mining area and development of heavy metal pollution index. App Water Sci. 2014;4:11-7.
- [CrossRef] [Google Scholar]
- Modulation of biochemical responses in rats following consumption of some herbalized Nigerian alcoholic drinks. Afr J Biomed Res. 2019;22:353-62.
- [Google Scholar]
- Temperature, pH, electrical conductivity, total dissolved solids and chemical oxygen demand of groundwater in Boji-BojiAgbor/Owa area and immediate suburbs. Res J Environ Sci. 2014;5:98-103.
- [CrossRef] [Google Scholar]
- Environmental implication of metal concentrations in soil, plant foods and pond in area around the Derelict Udege Mines of Nasarawa state, Nigeria. Bull Chem Soc Ethiopia. 2010;24:351-60.
- [CrossRef] [Google Scholar]
- Sustainable groundwater exploitation in Nigeria. J Water Resourc Ocean Sci. 2013;2:9-14.
- [CrossRef] [Google Scholar]
- Drinking water quality and public health of selected towns in South Western Nigeria. Water Qual Exp Health. 2013;6:13.
- [CrossRef] [Google Scholar]
- Assessment of water quality in Canaanland, Ota, Sothwest Nigeria. Agric Biol J North Am. 2011;7:15-26.
- [Google Scholar]
- Physicochemical properties of well, borehole and stream waters in Kubwa, Bwari Area Council, Federal Capital Territory, Nigeria. Electron J Environ Agric Food Chem. 2011;10:2296-304.
- [Google Scholar]
- Health risk assessment of heavy metals in soils and vegetables from wastewater irrigated area, Beijing-Tianjin city cluster, China. J Environ Sci. 2012;24:690-8.
- [CrossRef] [Google Scholar]